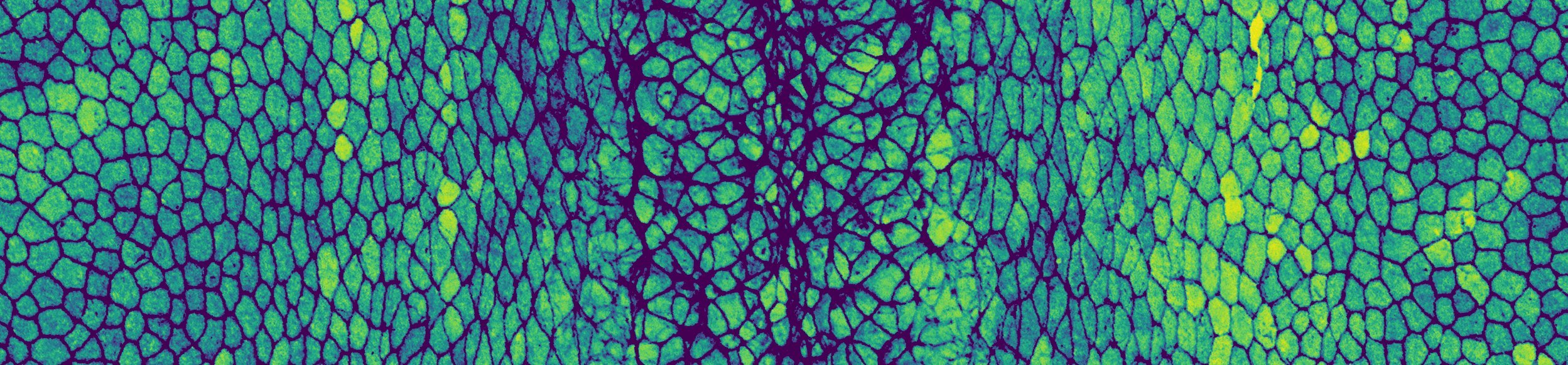
Mechanics of Morphogenesis:
The Biology and Engineering Behind the Mysteries of Embryonic Development.
Physics Matters! Biological processes play out in the physical world where work, energy, and kinetics dictate the shape and form of embryos and organs.
Our mission is two-fold, first, to expose the ways in which the environment, genome, cell biology, and mechanics are integrated during development, and second, to turn the principles of development into practical technologies for tissue engineering.
Our Group
-
Lance A Davidson, MSc, PhD
William Kepler Whiteford Professor and Director
-
Carsten Stuckenholz, MS, PhD
Research Scientist
-
Sommer Anjum
Graduate Student Researcher, Computational Modeling & Simulation
-
Yicheng Dong, MS
Graduate Student Researcher, Bioengineering
-
Geneva Masak
Graduate Student Researcher, Integrative Systems Biology
-
Benjamin McCutchan
Undergraduate Researcher, Bioengineering
-
Chloe Merino
Undergraduate Researcher, Biochemistry
-
Jing Yang
Graduate Student Researcher, Bioengineering
-
Rithik Rajasekar
Undergraduate Researcher,
Biological Sciences -
Yingli Wang
Undergraduate Researcher, Bioengineering